📝 Summary
Valence Bond Theory (VBT) explains the formation of bonds in coordination compounds, specifically how transition metals interact with ligands to create stable complexes. VBT focuses on orbital overlap, hybridization, and geometrical arrangements that help in predicting the properties of these compounds. Coordination compounds, characterized by a central metal ion and surrounding ligands, exhibit unique features like color and stability, and they play vital roles in fields such as medicine and catalysis. Understanding VBT is essential for grasping these phenomena.
- Valence Bond Theory in Coordination Compounds
- Understanding Coordination Compounds
- Valence Bond Theory: An Overview
- Hybridization in Coordination Compounds
- Coordination Numbers and Their Significance
- The Role of Ligands in Coordination Compounds
- Properties of Coordination Compounds
- Applications of Coordination Compounds
- Challenges and Future Directions
- Conclusion
- Related Questions on Valence Bond Theory in Coordination Compounds
Valence Bond Theory in Coordination Compounds
Valence Bond Theory (VBT) is a fundamental concept in chemistry that helps us understand the formation of bonds between atoms. Particularly in coordination compounds, VBT provides insight into how transition metals combine with various ligands, forming a stable complex. This article will delve into the essentials of Valence Bond Theory, its significance in coordination compounds, and how it aids in predicting the properties of these complexes.
Understanding Coordination Compounds
Coordination compounds, also known as coordination complexes, consist of a central metal atom or ion surrounded by molecules or ions called ligands. These ligands donate electron pairs to the metal, forming coordinate covalent bonds. Some key features of coordination compounds include:
- Central Metal Atom: Typically, a transition metal ion.
- Ligands: These can be anions or neutral molecules that donate electrons.
- Coordination Number: Number of ligands attached to the metal atom.
Examples of coordination compounds include hemoglobin, a complex of iron and protein, and [Cu(NH3)4]^2+, a copper complex with ammonia ligands.
Examples
For example, in the complex ion [Fe(CN)6]^{4-}, the iron atom (Fe) is the central metal, while the six cyanide ions (CN–) function as ligands.
Valence Bond Theory: An Overview
Valence Bond Theory was developed to explain how atoms form bonds, focusing on the idea that overlapping orbitals can create stronger bonds. In VBT, a bond forms when atomic orbitals from two atoms overlap to allow for the sharing of electron pairs. Importantly, it accounts for both ionic and covalent bonding. The key principles of VBT include:
- Orbital Overlap: Bonds are formed when atomic orbitals overlap, leading to electron sharing.
- Hybridization: The concept of hybridization plays a crucial role in coordination compounds.
- Bond Angles and Shapes: The geometry of the complex is determined by hybridization and the coordination number.
By examining the types of overlaps involved in chemical bonding, we can better understand the behavior of coordination complexes.
Hybridization in Coordination Compounds
Hybridization refers to the mixing of atomic orbitals to create new hybrid orbitals. Each hybrid orbital has properties that can explain the shapes and bond angles in coordination compounds. The two common types of hybridization in coordination compounds include:
- sp3 Hybridization: Forms four equivalent hybrid orbitals, often seen in tetrahedral complexes.
- d2sp3 Hybridization: Involves an octahedral arrangement, typical of many transition metal complexes.
Understanding hybridization helps in visualizing how ligands fit around the central metal ion in coordination compounds.
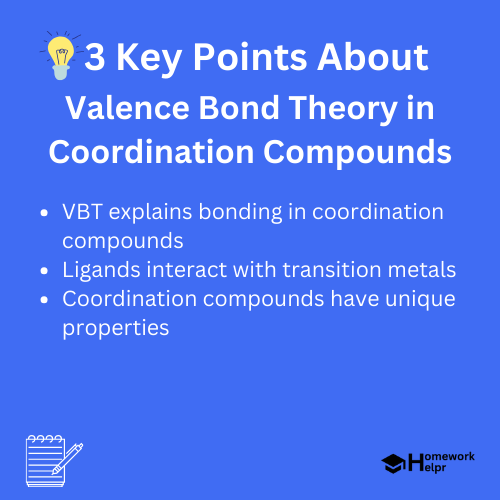
Coordination Numbers and Their Significance
The coordination number is a critical concept in coordination chemistry. It indicates the number of ligand donor atoms that surround the central metal ion. Different coordination numbers correspond to different geometrical arrangements. Common coordination numbers and their geometries are:
- 2: Linear geometry (e.g., [Ag(NH2)]2)
- 4: Tetrahedral or square planar geometry (e.g., [Ni(CO)4])
- 6: Octahedral geometry (e.g., [Co(NH3)6]^{3+})
The coordination number can influence various properties of the compound, such as stability, color, and reactivity.
Definition
Coordination Number: The total number of ligand atoms bonded to the central metal in a coordination complex.
❓Did You Know?
Did you know that hemoglobin, which enables our blood to transport oxygen, is an example of a coordination compound where iron is the central metal surrounded by complex protein ligands?
The Role of Ligands in Coordination Compounds
Ligands play an essential role in the formation and stability of coordination compounds. They can be classified based on the number of donor atoms they possess:
- Monodentate: Ligands with one donor atom (e.g., H2O, NH3).
- Bidentate: Ligands with two donor atoms (e.g., ethylenediamine).
- Polydentate: Ligands with multiple donor atoms (e.g., EDTA).
The denticity of a ligand significantly affects the geometry and stability of the coordination complex. For instance, bidentate ligands like ethylenediamine can form ring structures, enhancing stability.
Examples
In the coordination complex [Cu(EDTA)]^{2-}, the EDTA ligand acts as a polydentate, wrapping around the copper ion and forming a stable structure.
Properties of Coordination Compounds
Coordination compounds exhibit unique properties that set them apart from simple ionic or molecular compounds. Some of these properties include:
- Color: Many coordination compounds are colored due to d-d electron transitions.
- Magnetism: The presence or absence of unpaired electrons affects magnetic properties.
- Stability: The stability of a complex can vary based on ligand strength and metal ion.
For example, the complex [Cu(H2O)6]^{2+} appears blue due to electronic transitions, making it visually distinctive.
Applications of Coordination Compounds
Coordination compounds find widespread application in various fields due to their unique properties. Some notable applications include:
- Catalysis: Many industrial reactions utilize coordination complexes as catalysts.
- Medicine: Coordination compounds are used in medications, such as cisplatin for cancer treatment.
- Analytical Chemistry: Coordination complexes help in the detection and quantification of metal ions.
For instance, the complex ion [Fe(CN)6]^{3-} is commonly used in colorimetric analysis to detect the presence of cyanide due to its distinct color change.
Challenges and Future Directions
Despite their significance, the study of coordination compounds poses challenges, particularly regarding understanding their reaction mechanisms and stability. Advances in computational chemistry and spectroscopy are aiding researchers in unraveling these complexities.
Future research may focus on the design of new ligands to create more robust and selective coordination compounds for use in fields like medicine and environmental remediation.
Conclusion
Valence Bond Theory provides a comprehensive framework for understanding coordination compounds, emphasizing the importance of orbital overlap and hybridization. With various applications ranging from medicine to catalysis, the study of coordination compounds remains crucial in advancing scientific knowledge. By grasping the concepts covered in this article, students can appreciate the elegance of chemistry and its pervasive influence in our daily lives.
Related Questions on Valence Bond Theory in Coordination Compounds
What is Valence Bond Theory?
Answer: VBT explains how atoms form bonds.
What are ligands?
Answer: Molecules or ions that donate electron pairs.
What is hybridization in coordination compounds?
Answer: Mixing of orbitals to form new hybrid orbitals.
Why are coordination compounds important?
Answer: They are used in medicine and catalysis.