📝 Summary
Molecular Orbital Theory (MOT) is a core concept in chemistry that elucidates how atoms bond to form molecules. Unlike older theories, MOT treats electrons as distributed over the entire molecule within molecular orbitals that result from the combination of atomic orbitals. This theory helps predict a molecule’s structure, stability, and properties, including its magnetic characteristics, which are determined by the presence of unpaired electrons. Understanding MOT is pivotal for grasping fundamental chemical reactions and interactions, enhancing students‚Äô knowledge of elemental and compound properties.
Molecular Orbital Theory
Molecular Orbital Theory (MOT) is a fundamental concept in chemistry that explains how atoms bond together to form molecules. This theory suggests that electrons in a molecule are not assigned to individual atoms but are instead spread over the entire molecule. Understanding MOT allows students to predict the structure, stability, and properties of various chemical compounds.
At the heart of MOT are molecular orbitals, which are the regions in a molecule where electrons are likely to be found. These orbitals can be formed by the combination of atomic orbitals from the bonding atoms. The bonding between atoms creates new orbitals known as bonding and antibonding molecular orbitals. This theory provides a more comprehensive understanding than the older Valence Bond Theory.
Formation of Molecular Orbitals
The formation of molecular orbitals occurs through the linear combination of atomic orbitals (LCAO). When two atomic orbitals combine, they form *bonding* and *antibonding* molecular orbitals. Bonding molecular orbitals are lower in energy and lead to increased stability, while antibonding orbitals are higher in energy and can destabilize a molecule.
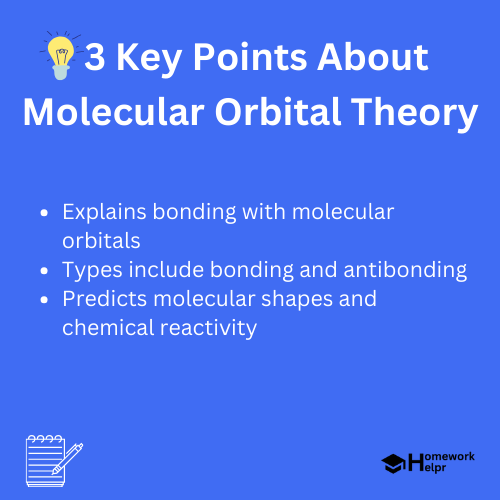
- Bonding Orbital: Formed when two atomic orbitals combine constructively, resulting in increased electron density between the nuclei.
- Antibonding Orbital: Formed when two atomic orbitals combine destructively, leading to decreased electron density between the nuclei.
For example, when the hydrogen (H) atoms bond to form H2, their 1s atomic orbitals overlap, creating a bonding orbital that holds the two hydrogen nuclei together. Conversely, an antibonding orbital with a star (σ*) configuration would pose a barrier to this bonding.
Examples
When two hydrogen atoms bond, their 1s orbitals combine into one bonding orbital (σ) and one antibonding orbital (σ*).
Types of Molecular Orbitals
Molecular orbitals are generally divided into two categories: *bonding* orbitals and *antibonding* orbitals, as mentioned. However, they can further be classified depending on the types of atoms involved and their energies.
- Sigma (σ) Orbitals: Formed from the end-to-end overlap of atomic orbitals.
- Pi (π) Orbitals: Formed from the side-to-side overlap of orbitals, present in double and triple bonds.
The energy levels and distributions of these orbitals influence the properties of molecules significantly. For example, molecules with filled bonding orbitals are generally stable, while those with filled antibonding orbitals can be reactive.
Examples
In a molecule like H2, there is one bonding σ orbital filled with electrons, leading to a stable diatomic molecule.
Electron Configuration in Molecules
Just as electrons fill atomic orbitals according to specific rules, they fill molecular orbitals based on their energy levels. The key principles governing this filling include:
- Pauli Exclusion Principle: No two electrons can have the same set of quantum numbers within a molecule.
- Aufbau Principle: Electrons occupy the lowest energy orbitals first.
- Hund‚’ Rule: When filling degenerate orbitals, electrons will occupy empty orbitals singly before pairing up.
According to these principles, the electron configuration of a diatomic molecule like oxygen (O2) can be represented as follows:
σ1s2 σ*1s2 σ2s2 σ*2s2 σ2p2
Definition
Degenerate: Refers to orbitals that have the same energy level.
Magnetic Properties and Molecular Stability
One fascinating application of molecular orbital theory lies in predicting the magnetic properties of molecules. Compounds with unpaired electrons in their molecular orbitals exhibit paramagnetism, meaning they will be attracted to a magnetic field. Conversely, molecules with all paired electrons are diamagnetic and are repelled by magnetic fields.
❓Did You Know?
Did you know that oxygen (O2) is paramagnetic due to the presence of two unpaired electrons in its molecular orbitals?
The stability of a molecule can be analyzed by calculating its bond order, which indicates the strength of the bond. The bond order is calculated using the formula:
Bond Order = $frac{(N_b – N_a)}{2}$
Where Nb is the number of electrons in bonding orbitals, and Na is the number of electrons in antibonding orbitals. A higher bond order signifies a stronger bond.
Examples
For the O2 molecule, which has 10 electrons in bonding orbitals and 6 in antibonding orbitals: Bond Order = $frac{(10 – 6)}{2} = 2$
Importance of Molecular Orbital Theory
Molecular Orbital Theory is crucial for understanding various aspects of chemistry, including:
- Predicting Molecular Shapes: MOT offers insights into molecular geometry and angles.
- Understanding Chemical Reactivity: It helps explain why certain molecules react in specific ways based on their molecular structures.
- Analyzing Spectra: The transitions of electrons between molecular orbitals give rise to unique spectral properties.
For instance, knowing the molecular orbitals of complex ions can help predict if they will absorb light at specific wavelengths, crucial in fields like photochemistry.
Definition
Photochemistry: The study of chemical reactions that involve light.
Conclusion
In summary, Molecular Orbital Theory provides a comprehensive framework for understanding the bonds between atoms in molecules. By recognizing that electrons are distributed across molecular orbitals rather than localized, students can better predict molecular properties and behaviors. Through its application in various fields of chemistry, MOT allows learners to delve more profoundly into the fascinating interactions that govern chemical reactivity and stability.
Exploring Molecular Orbital Theory leads to enhanced knowledge of elemental and compound properties, enriching students’ understanding of chemistry and its applications in real life. Whether in laboratory work or theoretical exploration, the principles of MOT remain essential for every budding scientist.
Related Questions on Molecular Orbital Theory
What is Molecular Orbital Theory?
Answer: It explains atomic bonding in molecules.
How are molecular orbitals formed?
Answer: Through linear combination of atomic orbitals.
What is the significance of bond order?
Answer: It indicates bond strength and stability.
How does MOT relate to magnetism?
Answer: It predicts paramagnetism and diamagnetism in molecules.